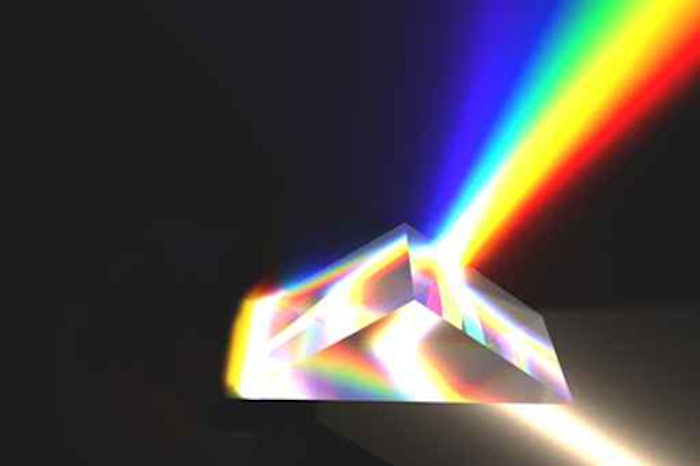
Protecting the skin from photodamage is the single most important measure an esthetician can emphasize in order to help prevent skin cancer and slow down the aging process in clients. To use sunscreens effectively requires a basic understanding of light energy, how light interacts with skin, and how to select and use sunscreens. Don’t panic, throw up your hands and run screaming because these concepts are quite simple and enjoyable to learn, and you will use them for the rest of your professional life in a variety of situations, from sunbathing to using laser equipment.
The basic physics of light
Many children have asked parents, “What is light?” only to receive the answer, “It is just light,” or “Nobody really knows.” In most cases, the child stopped there and waited for someone to come along and provide a better answer. At present, people are still in the dark when it comes to understanding light. Keeping that thought in mind, take a look at what is known about it, and don’t worry, because when all of this has been explained, you will understand a lot more about the physics of light and will be able see a small bit of the incredible beauty of the universe.
Light is energy. Light can be made only by using some form of energy to produce it. The sun is the major source of light during the day, and electricity is the major source at night, since moonlight is reflected sunlight. So, how is light actually made? Photons need to be produced. You may ask, “What in the world are photons?” A photon is the minimum bundle or capsule of energy needed to sustain the electromagnetic phenomenon at a particular frequency. Because a photon is a small packet of pure energy, it’s a tiny, tiny particle. Light is made up of these photon packets, and there are gazillions of them in a single ray of light.
Notice the word “electromagnetic” in the definition; it means that light has both an electric component and a magnetic component—that is why it is known as electromagnetic energy. Light travels in a straight line, but it moves up and down and in and out while it travels. A very interesting aspect of electromagnetic energy is that it travels as a wave with the components at right angles to each other. Figure 1 displays this relationship. Try to visualize this relationship by picturing a person walking with one arm straight up and the other arm held straight out to one side. The arm that is straight out is the electrical component, and the one that is straight up is the magnetic component.
Is the photon traveling in this wave pattern, carrying energy, or is energy shifting between electric andmagnetic forces in very discrete quantum of energy? This is the single most important bit of information about the photon: The energy of the photon is an exact amount known as a quantum, and is measured in electronvolts (eV). The energy in a single photon may be calculated by using the following expression, which is included not only for the budding scientists among estheticians, but also for those who have had the good fortune to study physics. For the rest, you can easily learn this and will then know how all these pieces of science relate.
The energy E, frequency f and wavelength λ of a photon are related by: E (energy) = h (Planck’s constanta) x f (frequency of light) or E = hf.
This formula can also be used and will provide the same result: E (energy) = h (Planck’s constant) x c (speed of lightb)/λ (wavelength of light) or E=hc/λ.
This can be made easier if h is multiplied by c to get the wavelength of 1,240 nanometers (nm), then all that is left to find a specific energy in eV for a photon in a particular wavelength is to divide 1,240 by that wavelength and the answer is available in eV. Here is an example: Find the energy of a photon with a λ of 400: E = 1,240 nm/λ = 1,240 nm/400 nm = 3.101 eV.
The main fact to learn from this equation is that the energy in a photon is discrete, directly related to the frequency of the wave and inversely related to the wavelength. Before giving up, this is important because the color of light depends on the wavelength of light. Blue light has a smaller wavelength than red light and, therefore, it has more energy. In Figure 2, the full range of the electromagnetic spectrum is shown with wavelengths of visible light clearly illustrated. For this article, visible light should be of less interest than ultraviolet light (UV), which packs a tremendous wallop. When the interaction of photons with the skin is covered, this will not only become clearer, but you will also understand why it is important to have this basic knowledge.
From radio waves to gamma rays
In Figure 2, the full range of the electromagnetic spectrum can be seen, from large radio waves to very small gamma rays. Radio waves can be more than one kilometer long, which is longer than several football fields arranged end to end, while gamma rays can be as small as one-millionth of a nanometer. Only a small part of the full electromagnetic spectrum can be seen; the range of wavelengths between 400–700 nm is called visible light. Below 400 nm is the range of UV light. The shorter the wavelength, the more energy the photon carries. Throughout the years, UV light was classified into separated wavelengths to denote various energy levels.
UV light is electromagnetic energy that ranges from approximately 160–400 nm. It is usually separated into three divisions:
1. UVA: 315–400 nm
2. UVB: 280–315 nm
3. UVC: 280– approx. 160 nm.
UVA, UVB and UVC are simply different wavelengths of UV energy. UVA is more commonly known as long wave or black light; UVB can be called medium wave; and UVC refers to short wave or germicidal. UVC does not reach the Earth because it is absorbed in the outer atmosphere, so this article will not cover it. It is the energy in the UVA and UVB range that affects the skin and produces so many problems. It is important to understand that these photons with various energy levels can enter the skin and interact with critical biochemical molecules in the skin.
How UV light interacts with skin
When light shines on the skin, several processes take place. Light can be reflected, scattered, transmitted into skin and then remitted—that is, sent back out to the surface. All of these processes have an effect on how much energy enters the skin and is able to react with the tissue. First, how light interacts with the skin’s surface will be reviewed, since this is the entrance point into the skin. Figure 3 is a diagram of reflected, transmitted and remitted light. Light first strikes the surface, and it is here that sunscreen is applied on exposed skin. The angle that the rays strike the skin has a major effect on the amount of light energy that is able to enter it. This can also be seen in Figure 4, which shows various amounts of sunlight at different times of the year. Also, notice in the figure that UVB is attenuated going through window glass, but attenuated even more when passing through auto glass. The take-home message here is be careful of sun exposure two hours before and two hours after noon.
Anything that reduces the light entering the skin will also reduce the potential damage from the UV rays. Before the 1950s, many teenage girls wore pancake makeup that acted as both a scattering agent and a sunblock. Even though they had no way of knowing this, they where protecting their skin from UV radiation damage. The whole purpose of sunscreen is to prevent or markedly reduce the effects of UV radiation on skin. After entering the skin, the UV light can react with the biochemicals in the skin. At this point, the domain of photochemistry is entered—an area that few dare to tread. Yes, it is a complex topic, but if you have an understanding of the basic principles of photochemistry, you have all the knowledge you need to use sunscreens both effectively and wisely.c
Essential photochemistry
Photochemistry covers many fields, but the branch of molecular photochemistry needed for this article involves how photons interact with organic matter. Even further, the focus on light will be limited to a wavelength between 200–700 nm, which covers both UV and visible electromagnetic radiation. You learned earlier in this article that, in the physics of light, photons travel in a wave and have both an electric and magnetic component, and that light travels in these waves at different frequencies. At this point, organic molecules will be discussed in order to get a bit of information to tie light interaction to the skin.
All organic molecules have energy—more specifically, bond energy—the energy required to hold one atom to another to form the molecule.d As a result, some of these molecules and their composite atoms are vibrating at set frequencies, or oscillations. Some organic molecules have dipole oscillations within their atomic structures, and those molecules in the skin that can interact with light have dipole oscillations that match the oscillations in light waves. Only those molecules that have identical dipole oscillations of the light wave can absorb the energy from the light. That phenomenon is called resonance. In general, only organic molecules that are unsaturated can absorb UV light higher than 220 nm. This list includes both cyclic (one ring) or polycyclic (more than one ring) compounds, such as purine and pyrimidine bases in deoxyribonucleic acid (DNA).e It includes the cyclic amino acids tyrosine, tryptophan and phenylalanine; and the noncyclic amino acids cytosine and cysteine. The biochemicals melanin and hemoglobin are also very important. When UV light interacts with the aforementioned compounds, energy is transferred from the light via a photon and passed to the organic molecule, either temporarily or permanently changing that molecule.
When a molecule absorbs a photon, it is electrically excited. This is an unstable state, and the molecule must return rapidly to its pre-excited state to survive. It can do this by four possible mechanisms.
- Give up the energy as heat. Heat is a form of energy that is easily transfered from one object to another, or just radiated into the air. The loss of heat in molecules means it returns to lower energy, known as the ground state.
- Give up the photon as light. When molecules do this, the light emitted is always a lower energy than what was initially absorbed. This light is called emission fluorescence, and it is a technology used by many branches of science to study everything from simple measurements in blood to quantum mechanical exploration.f
- Undergo a permanent structural change in the molecule. This structural change allows the molecule to retain the extra energy from the photon, but a new molecule is formed. Some of these molecules are quite harmful to the body.g
- Form a new molecule. The excited molecule can react with an appropriate molecule nearby and form a new molecule.
Next, let’s take a look at a few of the adverse reactions from UVB and UVA.
UVB and adverse skin reactions
An excess exposure to UVB radiation can cause sunburn and some forms of skin cancer. In humans, eye and immune system damage are common effects of sunlight. UV photons harm the DNA molecules of living organisms in different ways.1 In one common form of UV damage in DNA, adjacent cytosine bases bond with each other, forming a dimer and causing the DNA molecule to change into an abnormal shape so it can no longer function properly. See Figure 5. This is bad enough, but the big problem occurs when the DNA code must be replicated in this strand of DNA. The enzyme polymerase will read the dimer as “AA” and not the original “CC.” This will cause the DNA replication mechanism to add a “TT” on the growing strand rather than a “GG.”h Thus, a mutation is produced known as a classical C-T mutation, which can result in cancer. An interesting aspect of this mutation is that it carries a UV signature commonly seen in skin cancers, and the research scientist can recognize it as being caused by the direct DNA from UVB. Malignant melanoma is believed to be caused by the indirect DNA damage (free radicals and oxidative stress). This can be seen from the absence of a UV-signature mutation in 92% of all melanoma. Figure 6 shows the depth of penetration of UVA, UVB, UVC and infrared into the skin.
UVA and adverse skin reactions
The major acute effects of UV irradiation on normal human skin are sunburn, inflammation, erythema, tanning, and local or systemic immunosuppression. UVA and UVB can both damage collagen fibers by fragmenting them and cross-linking them, both conditions that accelerate skin aging.2 UVA and UVB can destroy vitamin A in the skin, causing loss of proliferative cell control. UVA penetrates deeply into the skin, but it does not cause sunburn or erythema, which may lead the public to think it is harmless, but nothing is further from the truth. Although UVA may not damage DNA directly like UVB since it has less energy, it can generate highly reactive chemical intermediates, such as hydroxyl and oxygen radicals, which really can damage DNA.3 Some scientists associate the absence of UVA filters in sunscreens with the higher risk of melanoma found in individuals who use sunscreens.4–6
Melanin
Melanin is derived from the amino acid tyrosine. The two major forms of human melanin are eumelanin, a brown-black melanin or regular melanin; and pheomelanin, which is a red-brown melanin seen in individuals with red hair and freckles.i The production of melanin in human skin—known as melanogenesis—is stimulated by UVA and UVB, and produces a darker color of the skin, known as a tan. Tanning is true melanogenesis. UVB triggers CPD-DNA damage, increases melanin production and may cause a sunburn, both caused by the direct DNA damage. UVB is reduced by most sunscreens; however, it produces brown spots or moles, and contributes to aging.
UVA can cause preexisting melanin to be released from the melanocytes and activates the oxidation of melanin, causing an immediate tan to appear. Melanoma seems to be associated with UVA.7 Although UVA contains fewer energetic photons, there is more UVA in sunlight and more of it each season. Melanin is able to absorb 99.9% of UV radiation and then dissipates this energy as heat by a process of ultrafast conversion of the absorbed photon energy to heat. It is this property of melanin that makes it a very good photo-protectant.
Collagen and elastin
The dermis contains two proteins that turn over very slowly. Collagen has a half-life of 15–17 years, and elastin is even longer at 70 years. What does half-life mean? This term is best explained by an example. Let’s say that in one area of the skin there are 1,000 molecules of collagen (actually you have millions upon millions of molecules of collagen). At age 17, 50% of these molecules will have either disappeared, or have been replaced, but 500 original molecules are still there. At age 34, another 250 molecules will disappear or have been replaced, but 250 of the original molecules are still there. At age 51, there are 125 of the original molecules left and at age 68 there are 6.25 original molecules left. That means these molecules have been subjected to UV radiation continuously for 68 years. No wonder a person gets worn out with time. Do not underestimate the ravages of UV light on these long-lived connective tissue proteins. Only by reducing the insult from high-energy UV radiation can this damage be prevented or attenuated.
Sunscreens
Products that are designated as sunscreens are designed to prevent or markedly attenuate high-energy UV from entering the skin. The formulation, manufacturing and marketing of sunscreens is regulated by the U.S. Food and Drug Administration (FDA) since sunscreens are designated as over-the-counter (OTC) drugs. They can be inorganic physical UV blocking agents, or organic chemicals that absorb UV light at specific wavelengths.
Physical, inorganic blocking agents are usually finely powdered minerals, the most common ones are zinc oxide and titanium dioxide. They act to block UV radiation by reflecting or scattering the rays, although some absorption of UV does occur. They are not normally absorbed, so they tend to produce a white color on the skin. To prevent this, the particles are often reduced in size so they no longer reflect light; particles smaller than 200 nm generally will not be seen on the skin. It is thought that such small particles may be able to penetrate the skin, but one study shows this is not the case.8 Also, in 2006, the Australian government found that zinc oxide and titanium dioxide do remain on the surface and in the outer dead layer of the skin. Bottom line, zinc oxide and titanium dioxide are safe in nanoparticle sizes. They are used alone, in combination or combined with organic chemical sunscreens, which are designed to cover absorption of either UVA or UVB, although some will cover the spectrum of both, though not completely.
UVB and sunscreens. See UVB Sunscreens for the types and amounts of each required to be in a product to provide a given sun protection factor (SPF). When buying a sunscreen, advise clients to look on the label to see how much protection they will get from the sunscreen and help them locate the SPF designation. Keep in mind that this only results in protection from UVB radiation and understand that an SPF rating is only a relative protection from skin-reddening due to the sun’s UVB rays. Remember that no sunscreen will stop 100% of UVB, but if it is applied properly, you can depend on an SPF 15 filtering out 93% of UVB, while a product with an SPF 30 filters out 97%, but even a high SPF 50 will filter out only 98%. In some individuals, such as blue-eyed blonds and green-eyed redheads, even 2–4 % of noontime UVB can induce a sunburn.
UVA and sunscreens. Remember that an SPF value does not indicate any protection against UVA. Currently the United States does not have a requirement to test the effectiveness of UVA sunscreens, although four other countries do. See UVA Sunscreens for a full list of approved sunscreens. It does have pending a recommended method to test UVA sunscreens. The methods used are both in vivo, that is, on a live human, or in vitro, instrument type, (literally, in glass). The in vivo test is called the Persistent Pigment Darkening (PPD) method. A PPD score of 10+ is considered good. This test has flaws since it is difficult to relate to real-world sun exposure, and it only measures photon energy below 320 nm.
Australia uses an in vitro test to assay UVA protection based on the ability of a sunscreen preparation to reduce the transmission of UV radiation between a wavelength of 320–360 nm to less than 10% of the incoming light. Japan uses the PPD method, as well. Another method is the critical wavelength test based on the previously described method, but modified to yield a new measure of the breadth of UVA protection. In this test, the absorbance of the thin film of the sunscreen is scanned from 290 nm across the UV wavelengths until the sum reaches 90% of the total absorbance of the sunscreen in the UV region (290–400 nm). The wavelength at which the summed absorbance reaches 90% of total absorbance is defined as the critical wavelength and is considered to be a measure of the breadth of sunscreen protection.9–12
Photostability. The stability of sunscreens has become a problem in sun protection. When a sunscreen becomes altered by UV absorption and breaks down, it is no longer an effective sunscreen. This has become a serious problem for manufacturers and is known as photostability. Essentially, the task is to have effective sunscreens absorbing high-energy photons and getting rid of the energy without being destroyed. Although this is not an easy task, excellent progress has been made. A new generation of organic molecules has been developed that can absorb this energy and release it quickly and with self-destruction. This process takes advantage of a photochemical reaction known as fluorescence quenching.13
The ingredients used in photostabilizing sunscreens can be added to a formulation or combined with other sunscreens. For example, ecamsule, a broad-spectrum sunscreen (290–400 nm with a peak at 345 nm), doesn’t cover the entire UV spectrum, so it must be combined with other active sunscreen agents to ensure broad-spectrum UV protection. Ecamsule is a photostable organic UVA absorber, meaning it doesn’t degrade significantly when exposed to light. A broad-spectrum sunscreen with ecamsule, avobenzone and octocrylene significantly reduces the skin damage associated with UV exposure in humans. Octocrylene is one of the cinnamate group of chemicals that absorbs light with wavelengths from 250–360 nm and is a photostabilizer.
Choosing a sunscreen
The selection of a sunscreen should be based on a client’s Fitzpatrick Skin Type and their lifestyle. Consider also if they have an associated skin disease of any autoimmune disease. See Fitzpatrick Skin Types for type descriptions, as well as recommendations for sunscreen selection.
Make sure clients have both UVA and UVB protection, and, in addition, that they have photostabilizers in their sunscreens. Help them identify whether there is a critical wavelength listed and if some type of UVA protective factor is noted besides the SPF. Remind them that a SPF of 30 is not twice as protective as a SPF of 15 and that they should wear hats and cotton gloves.
The Skin Cancer Foundation recommends applying at least 2 tablespoons of sunscreen—the same amount used in testing (2 mg/cm2 of skin)—to exposed areas 30 minutes before sun exposure, and reapplying it every two hours or immediately after excessive sweating or swimming. At times, if you want to increase the protection, just do a double application after the first application is dry.
Notes on tanning beds
UVA radiation makes up most of the radiation in tanning beds, which is associated with malignant melanoma, the most deadly form of skin cancer. It is fairly well established that each of the three main types of skin cancer—basal cell carcinoma, squamous cell carcinoma and melanoma—is caused by sun exposure. People who visit a tanning salon at least once a month are 55% more likely to develop melanoma than those who don’t. Those who use sun lamps to tan while in their 20s have about a 150% higher risk of skin cancer than similarly aged women who do not use tanning beds.
You know that exposure to UV radiation induces two of the most common DNA mutations known in cellular biology. Cells have developed a number of repair mechanisms to counteract the DNA damage caused by UV radiation, but they are not 100% effective. Exposure to UV radiation has a detrimental effect on the immune system, causing changes in antigen presentation by Langerhans cells and macrophages, and reducing the activities of natural killer cells and T cells. Herpes simplex is a frequently seen infection after UV exposure. UVA is clearly associated with aging changes in the skin, as well as the development of skin cancer. Most aging changes you have learned about in the skin are due to UVA rays destroying collagen and connective tissue. One good way to cause premature aging, including wrinkles, sunspots and loss of skin elasticity, is to use tanning beds.
The best of all worlds
What is new in the world of sunscreens is the use of microcapsules to encapsulate sunscreen and prevent direct contact of the sunscreen agent with the skin. This would act to markedly reduce, or even prevent sensitization from the degradation of the sunscreen. By adding a photostablilizer, the best of all worlds could be possible.
In reality, no matter what sunscreen you use, some UV still gets through to the skin. That is why sunscreen is a vital part of a comprehensive sun protection program, along with seeking the shade between high sun intensity times of day and wearing sun-protective clothing, including a wide-brimmed hat and UV-blocking sunglasses.
FOOTNOTES
a. Planck's constant = 6.626 x 10-27 ergs
b. Speed of light = 299,792,458 meters per second (m/s)
c. NJ Turro's Modern Molecular Photochemistry (University Science Books, 1991) is the text I recommend about photochemistry. It is advanced and mainly for professionals or undergraduate physics students. Another good reference in photochemistry is The Science of Photomedicine (Plenum Press, 1982) edited by JD Regan and JA Parrish
d. Remember that a person's energy in food comes from the sun through plants using photosynthesis. The sun's energy is locked in the molecules of the plant, and sugar is one of these important plant molecules. When sugar is eaten, the molecule is broken down and the energy is released.
e. Three are four bases in DNA: adenine, thymine, guanine and cytosine.
f. Currently, my laboratory is using fluorescence to measure the state of skin glycation by measuring the content of pentosidine in the skin.
g. Many modern types of photochemical reactions are used today; an example is the bonding of artifical nails and dental cosmetic bonding.
h. DNA bases can only join as A to T and C to G. That is, adenine to thymine and cytosine to guanine.
i. A third melanin exists known as neuromelanin, which is found in nerve cells.
REFERENCES
1. Y Matsumu and HN Ananthaswamy, Toxic effects of ultraviolet radiation on the skin, Toxicology and Applied Pharmacology 195 (3) 298–308 (2004)
2. RM Lavker and K Kaidbey, The spectral dependence for UVA- induced cumulative damage in human skin, J Invest Dermatol 108 17–21 (1997)
3. KM Hanson, E Gratton and CJ Bardeen, Sunscreen enhancement of UV-induced reactive oxygen species in the skin, Free Radical Biology and Medicine 41 (8) 1205–1212 (2006)
4. C Xu, A Green, A Parisi and PG Parsons, Photosensitization of the Sunscreen Octyl p-Dimethylaminobenzoate b UVA in Human Melanocytes but not in Keratinocytes, Photochemistry and Photobiology 73 (6) 600–604 (2001)
5. J Knowland, EA McKenzie, PJ McHugh and NA Cridland, Sunlight-induced mutagenicity of a common sunscreen ingredient, FEBS Letters 324 (3) 309–313 (1993)
6. MA Weinstock, Do sunscreens increase or decrease melanoma risk: An epidemiologic evaluation, J of Invest Dermatol Symposium Proceedings 4 97–100 (1999)
7. NS Agar, GM Halliday, RS Barnetson, HN Ananthaswamy, M Wheeler and AM Jones, The basal layer in human squamous tumors harbors more UVA than UVB fingerprint mutations: a role for UVA in human skin carcinogenesis, Proc Natl Acad Sci USA 101 4954-4959 (2004)
8. J Lademann, H Weigmann, C Rickmeyer, H Barthelmes, H Schaefer, G Mueller and W Sterry, Penetration of titanium dioxide microparticles in a sunscreen formulation into the horny layer and the follicular orifice, Skin Pharmacol Appl Skin Physiol 12 247–256 (1999)
9. B Herzog, Prediction of Sun Protection Factors by Calculation of Transmissions with a Calibrated Step Film Model, J Cosmet Sci 53 11–26 (Jan–Feb 2002)
10. A Chardon, D Moyal and C Hourseau, Persistent pigment-darkening response as a method for evaluation of ultraviolet A protection assays. Sunscreens: Development, Evaluation, and Regulatory Aspects, Marcel Dekker, NY (1997)
11. JCIA Measurement Standard for UVA Protection Efficacy, Japan Cosmetic Industry Association 105 (1995)
12. BL Diffey, A method for broad-spectrum classification of sunscreens, Int J Cosmet Sci 16 47–52 (1994)
13. C Bonda. Research Pathways to Photostable Sunscreens, Cosmetic & Toiletries 123 49–60 (2008)